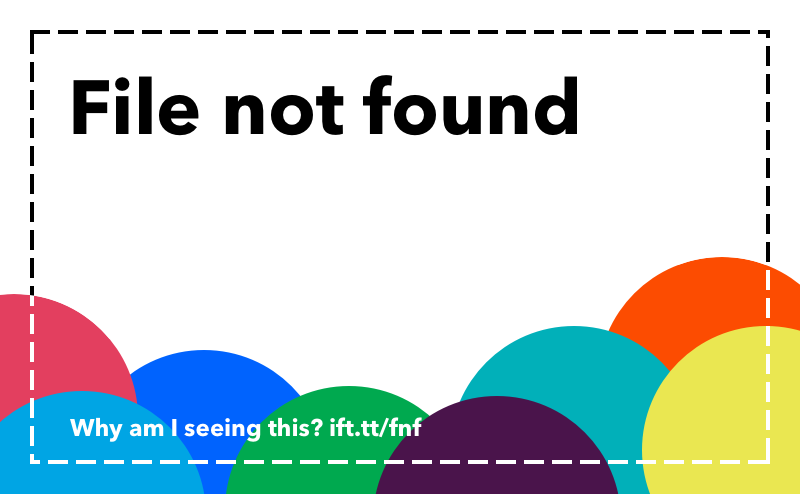
Nanoparticle ninja at large By day, I wield my Pharmaceutical Chemistry and Technology degree like a lightsaber, synthesizing reactions and making molecules do my bidding. By night, I don a cape and translate nanotech jargon into human speak for the non-lab-coat crowd. But let's be real, most of my time is spent in a pizza-fueled, cat-video-induced coma